Abstract
The respiratory system is not the only organ system affected by chronic obstructive pulmonary disease (COPD); muscle mass is also impacted. As skeletal and respiratory muscles deteriorate, ventilation, the severity of the illness, and clinical symptoms are all negatively impacted. According to recent studies, ultrasonography is a dependable and user-friendly method for determining the quadriceps contractile index (Qci) in patients with COPD.
Objective: Evaluate the Qci by ultrasound in patients with COPD and correlate with different clinical, 6-minute walk test (TM6), and ventilatory variables.
Methods: We conducted a prospective, observational study from 2021 to 2024. A total of sixty-one consecutive patients with spirometry-confirmed stable COPD were included after obtaining informed written consent. Demographic and clinical data, spirometric values, the modified Medical Research Council (mMRC) dyspnea score, TM6, COPD assessment test (CAT), Global Initiative for Chronic Obstructive Lung Disease (GOLD) classification 2023, and measurement of Qci were collected for analysis. This is a prospective study conducted at Constantine Regional Military University Hospital.
Results: The mean total quadriceps muscle thickness (Qthick) in our patients with COPD is 1.95 ± 0.85 cm. The mean Qci in our patients with COPD is 68 ± 9%. A negative correlation has been reported between Qci and the dyspnea mMRC, P = 0,010. Patients in group E according to GOLD 2023 constitute more than half of our cohort, representing 54.1% of cases. A negative correlation has been reported between Qci and the stage of GOLD 2023 classification, P = 0,010. In our study, a correlation between the Qci and spirometric data (FVC, FEV1) was objectified with P < 0,001 and FEV1/FVC P = 0,008. The average distance travelled in the TM6 test was 470 129.37 m. Our study has shown that the more EXdi increases, the distance patients travel increases (P = 0,001).
Conclusion: In patients with COPD, the Qci appears to be associated with airway obstruction, TM6, CAT score, as well as perception of dyspnea.
Keywords
chronic obstructive pulmonary disease, quadriceps contractile index, spirometry, dyspnea, ultrasound, 6-minute walk test, GOLD classification 2023
Abbreviations
COPD: chronic obstructive pulmonary disease; Qci: quadriceps contractile index; TM6: 6-minute walk test; mMRC: modified Medical Research Council; CAT: COPD assessment test; Qthick: total quadriceps muscle thickness; GOLD: Global Initiative for Chronic Obstructive Lung Disease; Atthick: total thickness of the anterior thigh; BMI: body mass index
Introduction
Chronic obstructive pulmonary disease (COPD) is a major public health problem and is a leading cause of chronic morbidity and mortality worldwide. Prevalence of COPD is difficult to assess because it requires patient cohorts representative of the entire population using spirometric measurements [1].
According to estimates, 384 million individuals worldwide suffered with COPD in 2010, and in 2015, the frequency was projected to be 11.7% (8.4–15.0%) [2].
According to the BOLD study, COPD is a significant public health issue and a primary cause of morbidity and mortality globally: COPD affects 10.1% of those over 40, and it is thought to cause three million deaths annually. The BREATHE study estimates that the prevalence of COPD in Algeria is 25% among smokers and 4% in the general population [3, 4].
Skeletal muscle dysfunction is an extrapulmonary complication of COPD, with muscle loss identified as a key determinant of disability [5] and an independent predictor of mortality [6]. In particular, the reduction of quadriceps strength that is associated with reduced exercise capacity [7], a reduced quality of life [8], increased use of health care [9], and mortality, regardless of airflow obstruction [10].
In patients with COPD, quadriceps strength is reduced by up to 30% compared to healthy peers of the same age [11].
Muscle atrophy of the thigh in COPD is a well-known clinical manifestation of COPD and is associated with systemic inflammation, chronic hypoxia, and low testosterone levels [12].
Exogenous oxidative stress is brought on by exposure to air pollution, biomass, and cigarette smoke; endogenous oxidative stress is brought on by inflammation and cell activation in the lungs, and a decrease in antioxidants causes oxidative stress to rise in the lungs. This plays a major role in the pathophysiology of COPD, how it develops, and the elevated risk of acute exacerbations. Chronic inflammation, a reduction in the anti-inflammatory effects of corticosteroids, accelerated lung aging, peripheral respiratory tract fibrosis, and an excess of mucus are all consequences of increased oxidative stress in the lungs of patients with COPD [13]. Oxidative stress plays an important role in the pathophysiology of COPD [14, 15]. According to certain research, the severity of COPD is correlated with oxidative stress, which can result in medical comorbidities and muscular dysfunction [16].
Although atrophy can affect all types of quadriceps fibers [17], type IIx fibers appear to be more frequently affected [18] even in the absence of severe airflow obstruction in COPD [19].
Changes in muscle fiber typography observed in the quadriceps do not affect muscles of the upper limbs, such as the bicep and deltoid [20, 21]. The idea that only some peripheral muscles have variations in the percentage of fiber types suggests that local factors (inflammation, oxidative stress, etc.) are responsible for these variations. By contractility, patients with COPD have a decrease in muscle strength due to quadriceps atrophy and decreased muscle fiber size [7, 22, 23]. In these patients, the decrease in quadriceps strength is proportional to muscle mass, suggesting that the contractile device was preserved [23]. In addition, in vitro experiments on the contractility of isolated quadriceps muscle bundles from patients with COPD and healthy controls confirm this hypothesis [24].
A number of studies have confirmed the reliability of ultrasound to measure quadriceps muscle size for patients with COPD [25, 26]. Ultrasound evaluation of the diaphragm and rectus femoris can be used as a marker to assess skeletal muscle dysfunction in COPD. Indeed, the diaphragmatic function and total thickness of the quadriceps muscle are reduced in patients with COPD [27, 28].
More recent studies have reported a significant reduction in the cross section of the rectus femoris (QCSA) in patients with COPD compared to healthy subjects [29–33]. This reduction is about 25% compared to the average values observed in healthy individuals [25]. In addition, a significant decrease of total quadriceps muscle thickness (Qthick) was observed in COPD patients [31, 33–36].
Ultrasound assessment of the quadriceps contractile index (Qci) is also feasible in patients with COPD. This index correlates with the severity of the disease, clinical symptoms, history of exacerbation, and contractility of diaphragmatic muscles. Thus, the Qci could be a new tool to assess the severity of COPD in this population [29]. The right femoral ultrasound can also predict impaired exercise tolerance in COPD patients [37]. Finally, in acute exacerbation hospitalization, a smaller quadriceps muscle size measured by ultrasound is associated with an increased risk of unplanned readmission or death [30].
Methods
This is a prospective descriptive observational study conducted in the HMRUC’s Department of Pulmonology, 61 patients participated in the study. According to the Global Initiative for Chronic Obstructive Lung Disease (GOLD) criteria:
Inclusion criteria: All patients with COPD over 40 years of age and in stable condition.
Exclusion criteria: Restrictive respiratory pathology, progressive cardiovascular disease, and recent surgery (less than 3 months).
A cross-sectional study design was used. All participants underwent ultrasonographic evaluation and other measurements (pulmonary function). This study was approved by the medical ethics committee of Constantine Regional Military University Hospital. The objective and content of the study were explained verbally as well as in written documents to the participants. Written consent was obtained after the subjects were informed that they could decide whether to participate based on their own free will and that their privacy would be reasonably protected.
The ultrasound examination was performed by a single specialized and trained professional using a TOSHIBA device, model SSA-370A PowerVision 6000.
To assess the echographic parameters of the quadriceps in a patient in supination position with the leg reclined, the linear probe is placed perpendicular to the longitudinal axis of the thigh at the most anterior central part of the femur bone, in an axis perpendicular to the skin.
Before the acquisition of images, patients are asked to perform contraction-relaxation maneuvers to delimit the muscular septa to separate the vastus intermedius and rectus femoris muscle. Three comparative measurements will be made, all in the state of relaxation (Figure 1) [38, 39].
Total quadriceps muscle thickness (Qthick) (in mm) (Figure 2).
Total thickness of the anterior thigh (Atthick) (distance between the skin and the anterior border of the femur) (in mm) (Figure 3).
The quadriceps contractile index (Qci)
Qci = Qthick/Atthick
All measurements will be made using a linear probe.
Figure 1: Ultrasound measurements of the quadriceps.
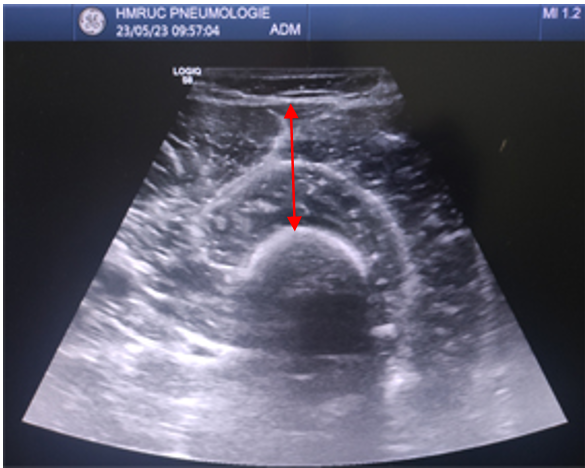
Figure 2: Total quadriceps muscle thickness (Qthick).
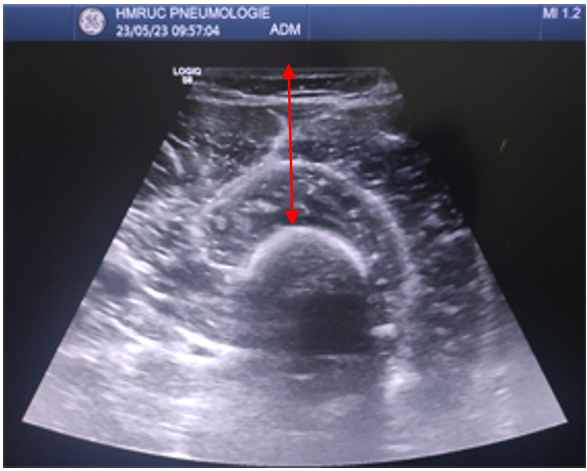
Figure 3: Total thickness of the anterior thigh (Atthick).
Other measurements
Spirometry was performed with a previously calibrated spirometer in accordance with the methods and criteria recommended by the American Thoracic Society and the European Respiratory Society [40]. The following parameters were measured: FVC, FEV1, and FEV1/FVC before and 15 min after inhalation of a bronchodilator (albuterol, 400 μg).
Dyspnea was measured with the modified Medical Research Council (mMRC) dyspnea scale [22], the degree of dyspnea ranging from 0 (no dyspnea) to 4 (very severe dyspnea). Patients were instructed to select the number that best represented their perception of dyspnea [41].
Height and weight were measured and the body mass index (BMI) was calculated. Obese patients (BMI 30 kg/m2) were excluded from the study.
Statistics analysis
Data was captured and analyzed using IBM SPSS 24. Many procedures of control at the time of entry have been established to avoid errors as much as possible. We have implemented the following statistical methods:
- Frequencies and percentages for qualitative data.
- The means, standard deviation, maximum, and minimum for quantitative data.
- The normality of quantitative variables was investigated by the Shapiro–Wilk test.
- Comparisons of summer averages performed by the Student’s t-test or ANOVA test according to the number of modalities of the variable.
- Comparisons between categorical or nominal variables were made by the Chi2-test or, where appropriate, by the Fisher’s exact test.
- The study of relationships between quantitative variables was analyzed by the Pearson or Spearman’s correlation coefficient as a function of the statistical distribution of variables.
The statistical tests used were considered significant when p = 0.05 (degree of significance).
Results
The population in our study is almost exclusively male, 1 female/60 male, sex-ratio = 0.017.
The majority of patients included in the study are in both age groups, 60–70 and 70–79, representing 73.8% of the total population (Figure 4). The average BMI is 24.12 ± 4.83 kg/m2, with extreme values ranging from 14.6–36.9 kg/m2.
Three groups of patients were identified according to smoking status:
- Ex-smokers: They represent the majority of our cohort with 73.8% of patients (n = 45).
- Active smokers: 22.9% of patients are active smokers (n = 14).
- Non-smokers: Only 3.3% of patients have never smoked (n = 2).
The majority of our patients were classified grade 1 and grade 2 according to mMRC (Figure 5). A negative correlation has been reported between Qci and the dyspnea mMRC, ANOVA F = 3,640, P = 0,010 (Figure 6).
The mean Qthick in our patients with COPD is 1.95 ± 0.85 cm. The mean Qci in our patients with COPD is 68 ± 9%.
The median COPD assessment test (CAT) score of our population is 14 ± 6.75 with extremes ranging from 4–30. 67.2% of our patients have a CAT score greater than or equal to 10, Qci was significantly correlated with CAT score (P = 0,001).
Patients in group E according to GOLD 2023 constitute more than half of our cohort, representing 54.1% of cases (Figure 7). A negative correlation has been reported between Qci and the stage of GOLD 2023 classification, ANOVA F = 5,03, P = 0,010 (Figure 8). In our study, a correlation between the Qci and spirometric data (FVC, FEV1) was objectified with P < 0,001 and FEV1/FVC P = 0,008 (Tables 1 and 2).
A negative correlation has been reported between Qci and the stage of severity of FEV1, ANOVA F = 3,47, P = 0,022 (Figure 9).
The average distance travelled in this test was 470 129.37 m. Our study has shown that the more EXdi increases, the distance patients travel increases (P = 0,001) (Figure 10).
Figure 4: Distribution of patients by age group.
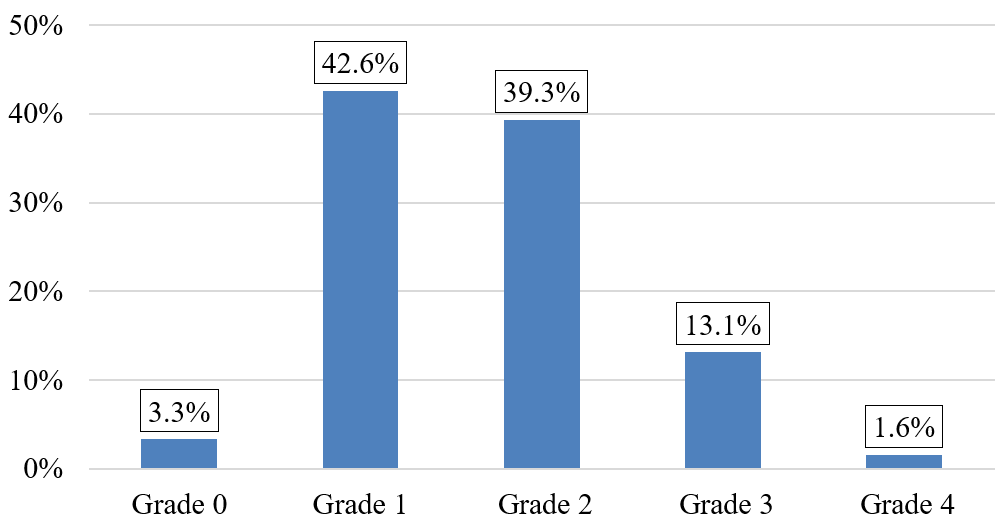
Figure 5: Patient distribution according to mMRC scale.
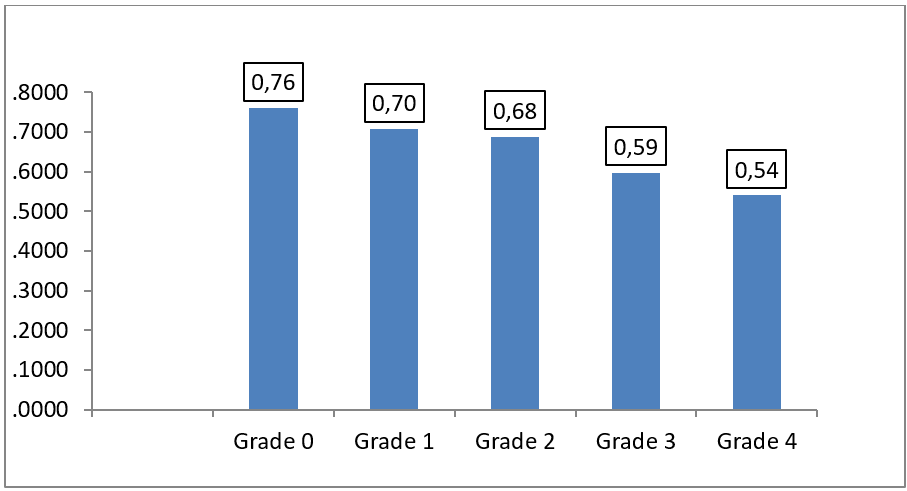
Figure 6: Mean of the Qci according to the severity of the dyspnea severity according to MMRC. ANOVA F = 3,640, P = 0,010.
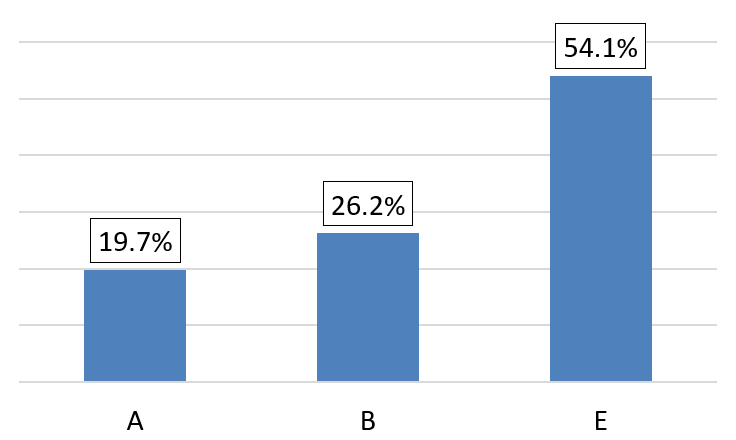
Figure 7: Patient distribution according to GOLD 2023 classification.
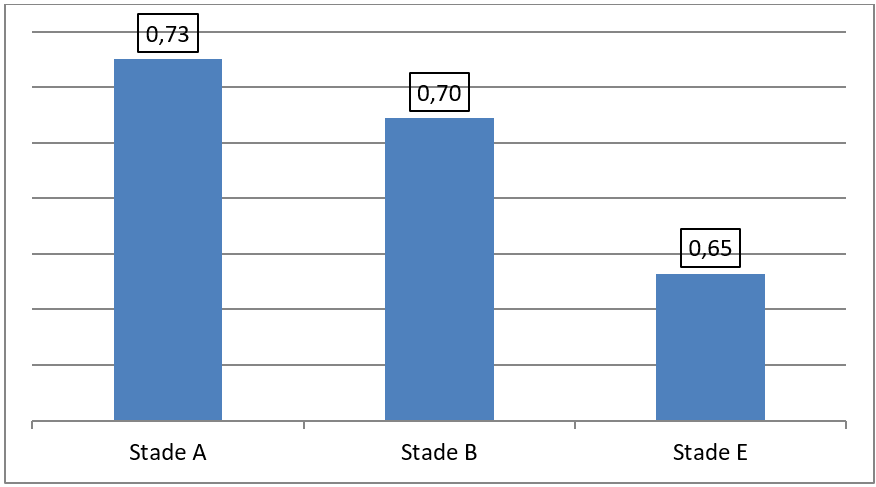
Figure 8: Mean of the Qci according to different stages of GOLD 2023 classification. ANOVA F = 5,03, P = 0,01.
| Average | Standard |
FVC |
FVC pré (L) | 3,52 | 0,92 |
FVC post (L) | 3,63 | 0,90 |
FEV1 |
FEV1 pré (L/s) | 1,91 | 0,69 |
FEV1 post (L/s) | 2,00 | 0,71 |
FEV1/FVC (%) |
FEV1/FVC pré (%) | 53,57 | 10,56 |
FEV1/FVC post (%) | 54,74 | 11,73 |
FEV1 post – FEV1 pré (L/s) | 110 | 90 |
(FEV1 post – FEV1 pré) ⁄ FEV1 pré (%) | 6,7 | 5 |
Table 1: Spirometric characteristics of the studied population.
Variable | Qci (%) |
Pearson correlation coefficient | P value |
FVC pré (l) | 0,496 | <0,001 |
FEV1 pré (l/s) | 0,514 | <0,001 |
FEV1/FVC pré (%) | 0,334 | 0,008 |
Table 2: Correlation between Qci and spirometric results.
Figure 9: Mean of the Qci as a function of severity of FEV1. ANOVA F = 3,471, P = 0,022.
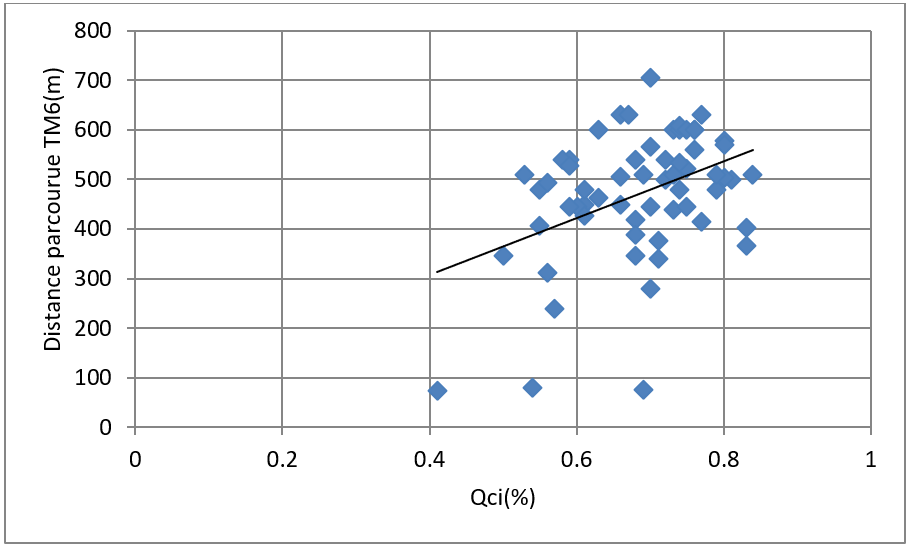
Figure 10: Correlation between Qci and distance travelled TM6 (m). Pearson correlation coefficient = 0,410, P = 0,001.
Discussion
In the present study, we found a significant positive correlation between Qci and spirometric data. These results are in line with those reported by Maynard-Paquette et al. [29] who noted that Qci was significantly correlated with FEV1 (p = 0.001) but not with age.
In our cohort, we also observed a significant negative correlation between Qci and impact of COPD on life (CAT score) (P = 0,001). These results corroborate those of Maynard-Paquette et al. [29] (p = 0,002).
Maynard-Paquette et al. [29] used this method in a prospective cohort study. They stated that in patients with COPD, an ultrasound assessment of the Qci is possible and is related to the severity of the disease, clinical symptoms, and history of exacerbations. The same findings were observed in our study, a negative correlation has been reported between Qci and the stage of severity of FEV1, ANOVA F = 3,47, P = 0,022.
However, further studies are needed to better determine its potential role as a prognostic marker in this population.
Conclusion
The quadriceps ultrasound has proven to be a valuable tool for assessing lower limb morphology and function in patients with COPD. It is therefore recommended to systematically integrate this examination in the management of patients, especially those with dyspnea symptoms. Ultrasound, a non-invasive technique, could be used as an early detection tool to identify patients at risk for muscle dysfunction even in the early stages of COPD.
In summary, this study shows that the lower the contractility of the quadriceps in patients with COPD, the lower the quality of life and exercise capacity. A decreased Qci may be a factor in reduced exercise capacity in patients with COPD. Quadriceps ultrasound can help physicians develop better treatment strategies, which requires more research and applications.
Finally, it is recommended that investment be made in the acquisition and implementation of portable ultrasound devices in health care facilities, particularly those receiving patients with COPD. The availability of these devices, as well as training health care teams in their use, would facilitate access to this diagnostic tool and make it available in intensive care units and rehabilitation centers.
Ethical Compliance
The research experiments conducted in this article with animals or humans have been approved by the ethics committee and the authorities responsible for our research organizations, in accordance with all guidelines, regulations, legal and ethical standards required for humans or animals.
Acknowledgements
Our gratitude to Professor Djeghri Yacine who supported this work. Thank you to all the authors who have contributed to the literature review, critically reviewed the manuscript for its important intellectual content, approved the final version to be published and agreed to act as guarantors of the work.
Authors Contributions
Meridj A: Designed and developed the analysis, wrote the article; Belaala R: Collected data; Djeghri Y: Conducted the analysis.
References
- Chabot F, Zysman M, Guillaumot A, et al. La bronchopneumopathie chronique obstructive [Chronic obstructive pulmonary disease]. Bull Acad Natl Med. 2019;203(1-2):63-71.
- Adeloye D, Chua S, Lee C, et al. Global and regional estimates of COPD prevalence: Systematic review and meta-analysis. J Glob Health. 2015;5(2):020415.
- Buist AS, Vollmer WM, McBurnie MA. Worldwide burden of COPD in high- and low-income countries. Part I. The burden of obstructive lung disease (BOLD) initiative. Int J Tuberc Lung Dis. 2008;12(7):703-08.
- Khelafi R, Aissanou A, Tarsift S, et al. Épidémiologie de la bronchopneumopathie chronique obstructive dans la wilaya d’Alger [Epidemiology of chronic obstructive pulmonary disease in Algiers]. Rev Mal Respir. 2011;28(1):32-40.
- Casaburi R, Gosselink R, Decramer M, et al. Skeletal muscle dysfunction in chronic obstructive pulmonary disease. A statement of the American Thoracic Society and European Respiratory Society. Am J Respir Crit Care Med. 1999;159(4 Pt 2):S1-40.
- Schols AM, Broekhuizen R, Weling-Scheepers CA, et al. Body composition and mortality in chronic obstructive pulmonary disease. Am J Clin Nutr. 2005;82(1):53-59.
- Gosselink R, Troosters T, Decramer M. Peripheral muscle weakness contributes to exercise limitation in COPD. Am J Respir Crit Care Med. 1996;153(3):976-80.
- Simpson K, Killian K, McCartney N, et al. Randomised controlled trial of weightlifting exercise in patients with chronic airflow limitation. Thorax. 1992;47(2):70-75.
- Decramer M, Gosselink R, Troosters T, et al. Muscle weakness is related to utilization of health care resources in COPD patients. Eur Respir J. 1997;10(2):417-23.
- Swallow EB, Reyes D, Hopkinson NS, et al. Quadriceps strength predicts mortality in patients with moderate to severe chronic obstructive pulmonary disease. Thorax. 2007;62(2):115-20.
- Man WD, Soliman MG, Nikoletou D, et al. Non-volitional assessment of skeletal muscle strength in patients with chronic obstructive pulmonary disease. Thorax. 2003;58(8):665-69.
- Wüst RC, Degens H. Factors contributing to muscle wasting and dysfunction in COPD patients. Int J Chron Obstruct Pulmon Dis. 2007;2(3):289-300.
- Barnes PJ. Oxidative Stress in Chronic Obstructive Pulmonary Disease. Antioxidants. 2022;11(5):965.
- Scicchitano BM, Pelosi L, Sica G, et al. The physiopathologic role of oxidative stress in skeletal muscle. Mech Ageing Dev. 2018;170:37-44.
- Lage VKDS, de Paula FA, Dos Santos JM, et al. Are oxidative stress biomarkers and respiratory muscles strength associated with COPD-related sarcopenia in older adults? Exp Gerontol. 2022;157:111630.
- Sepúlveda-Loyola W, Osadnik C, Phu S, et al. Diagnosis, prevalence, and clinical impact of sarcopenia in COPD: a systematic review and meta-analysis. J Cachexia Sarcopenia Muscle. 2020;11(5):1164-176.
- Whittom F, Jobin J, Simard PM, et al. Histochemical and morphological characteristics of the vastus lateralis muscle in patients with chronic obstructive pulmonary disease. Med Sci Sports Exerc. 1998;30(10):1467-474.
- Gosker HR, Engelen MP, van Mameren H, et al. Muscle fiber type IIX atrophy is involved in the loss of fat-free mass in chronic obstructive pulmonary disease. Am J Clin Nutr. 2002;76(1):113-19.
- Seymour JM, Spruit MA, Hopkinson NS, et al. The prevalence of quadriceps weakness in COPD and the relationship with disease severity. Eur Respir J. 2010;36(1):81-88.
- Sato Y, Asoh T, Honda Y, et al. Morphologic and histochemical evaluation of muscle in patients with chronic pulmonary emphysema manifesting generalized emaciation. Eur Neurol. 1997;37(2):116-21.
- Gea JG, Pasto M, Carmona MA, et al. Metabolic characteristics of the deltoid muscle in patients with chronic obstructive pulmonary disease. Eur Respir J. 2001;17(5):939-45.
- Bernard S, LeBlanc P, Whittom F, et al. Peripheral muscle weakness in patients with chronic obstructive pulmonary disease. Am J Respir Crit Care Med. 1998;158(2):629-34.
- Hamilton AL, Killian KJ, Summers E, et al. Muscle strength, symptom intensity, and exercise capacity in patients with cardiorespiratory disorders. Am J Respir Crit Care Med. 1995;152(6 Pt 1):2021-031.
- Debigaré R, Côte CH, Hould FS, et al. In vitro and in vivo contractile properties of the vastus lateralis muscle in males with COPD. Eur Respir J. 2003;21(2):273-78.
- Seymour JM, Ward K, Sidhu PS, et al. Ultrasound measurement of rectus femoris cross-sectional area and the relationship with quadriceps strength in COPD. Thorax. 2009;64(5):418-23.
- Meridj A, Belaala R, Djeghri Y. Innovative Technique for Muscle Exploration in Patients With COPD. International Journal of Pulmonology and Disorders. 2024;3(1):1-4.
- Ramachandran P, Devaraj U, Patrick B, et al. Ultrasonographic assessment of skeletal muscle mass and diaphragm function in patients with chronic obstructive pulmonary disease: A case-control study. Lung India. 2020;37(3):220-26.
- Meridj A, Belaala R, Djeghri Y. Influence of COPD on the Diaphragm and Muscles of the Lower Limbs. J Pulmonol Respir Res. 2024;8(2):56-59.
- Maynard-Paquette AC, Poirier C, Chartrand-Lefebvre C, et al. Ultrasound Evaluation of the Quadriceps Muscle Contractile Index in Patients with Stable Chronic Obstructive Pulmonary Disease: Relationships with Clinical Symptoms, Disease Severity and Diaphragm Contractility. Int J Chron Obstruct Pulmon Dis. 2020;15:79-88.
- Greening NJ, Harvey-Dunstan TC, Chaplin EJ, et al. Bedside assessment of quadriceps muscle by ultrasound after admission for acute exacerbations of chronic respiratory disease. Am J Respir Crit Care Med. 2015;192(7):810-16.
- Nijholt W, Beek LT, Hobbelen JSM, et al. The added value of ultrasound muscle measurements in patients with COPD: An exploratory study. Clin Nutr ESPEN. 2019;30:152-58.
- Ramírez-Fuentes C, Mínguez-Blasco P, Ostiz F, et al. Ultrasound assessment of rectus femoris muscle in rehabilitation patients with chronic obstructive pulmonary disease screened for sarcopenia: correlation of muscle size with quadriceps strength and fat-free mass. Eur Geriatr Med. 2019;10(1):89-97.
- Ye X, Wang M, Xiao H. Echo intensity of the rectus femoris in stable COPD patients. Int J Chron Obstruct Pulmon Dis. 2017;12:3007-015.
- Shrikrishna D, Patel M, Tanner RJ, et al. Quadriceps wasting and physical inactivity in patients with COPD. Eur Respir J. 2012;40(5):1115-122.
- Cruz-Montecinos C, Guajardo-Rojas C, Montt E, et al. Sonographic Measurement of the Quadriceps Muscle in Patients With Chronic Obstructive Pulmonary Disease: Functional and Clinical Implications. J Ultrasound Med. 2016;35(11):2405-412.
- Seymour JM, Ward K, Raffique A, et al. Quadriceps and ankle dorsiflexor strength in chronic obstructive pulmonary disease. Muscle Nerve. 2012;46(4):548-54.
- Deng M, Liang C, Yin Y, et al. Ultrasound assessment of the rectus femoris in patients with chronic obstructive pulmonary disease predicts poor exercise tolerance: an exploratory study. BMC Pulm Med. 2021;21(1):304.
- Agyapong-Badu S, Warner M, Samuel D, et al. Anterior thigh composition measured using ultrasound imaging to quantify relative thickness of muscle and non-contractile tissue: a potential biomarker for musculoskeletal health. Physiol Meas. 2014;35(10):2165-176.
- Guerreiro AC, Tonelli AC, Orzechowski R, et al. Bedside Ultrasound of Quadriceps to Predict Rehospitalization and Functional Decline in Hospitalized Elders. Front Med (Lausanne). 2017;4:122.
- Miller MR, Hankinson J, Brusasco V, et al. Standardisation of spirometry. Eur Respir J. 2005;26(2):319-38.
- Hajiro T, Nishimura K, Tsukino M, et al. Analysis of clinical methods used to evaluate dyspnea in patients with chronic obstructive pulmonary disease. Am J Respir Crit Care Med. 1998;158(4):1185-189.